28/07/2011: Tiny flying machines inspired by nature will revolutionise surveillance work
EPSRC Press release
Tiny aerial vehicles are being developed with innovative flapping wings based on those of real-life insects.
Incorporating micro-cameras, these revolutionary insect-size vehicles will be suitable for many different purposes ranging from helping in emergency situations considered too dangerous for people to enter, to covert military surveillance missions.
Supported by the Engineering and Physical Sciences Research Council, world-leading research at the University of Oxford is playing a key role in the vehicles' development.
Dr Richard Bomphrey, from the Department of Zoology, is leading this research, which is generating new insight into how insect wings have evolved over the last 350 million years. "Nature has solved the problem of how to design miniature flying machines," he says. "By learning those lessons, our findings will make it possible to aerodynamically engineer a new breed of surveillance vehicles that, because they're as small as insects and also fly like them, completely blend into their surroundings."
Currently the smallest of state-of-the-art fixed-wing unmanned surveillance vehicles are around a foot wide. The incorporation of flapping wings is the secret to making the new designs so small. To achieve flight, any object requires a combination of thrust and lift. In manmade aircraft, two separate devices are needed to generate these (i.e. engines provide thrust and wings provide lift), this limits the scope for miniaturising flying machines.
But an insect's flapping wings combine both thrust and lift. If manmade vehicles could emulate this more efficient approach, it would be possible to scale down flying machines to much smaller dimensions than is currently possible.
"This will require a much more detailed understanding than we currently have of how insect wings have evolved, and specifically of how different types of insect wing have evolved for different purposes," Dr Bomphrey says. "For instance, bees are load-lifters, a predator such as a dragonfly is fast and manoeuvrable, and creatures like locusts have to range over vast distances. Investigating the differences between insect wing designs is a key focus of our work. These ecological differences have led to a variety of wing designs depending on the task needing to be performed. It means that new vehicles could be customised to suit particular uses ranging from exploring hostile terrain, collapsed buildings or chemical spills to providing enhanced TV coverage of sports and other events".
Dr Bomphrey and his team lead the world in their use of both cutting-edge computer modelling capabilities and the latest high-speed, high-resolution camera technology to investigate insect wing design and performance.
Key to the work is the calculation of air flow velocities around insect wings. This is achieved by placing insects in a wind tunnel, seeding the air with a light fog and illuminating the particles with pulsing laser light - using a technique called Particle Image Velocimetry.
The team's groundbreaking work has attracted the attention of NATO, the US Air Force and the European Office of Aerospace Research and Development. The research is expected to produce findings that can be utilised by the defence industry within 3-5 years, leading to the development and widespread deployment of insect-sized flying machines within 20 years.
"This is just one more example of how we can learn important lessons from nature," says Dr Bomphrey. "Tiny flying machines could provide the perfect way of exploring all kinds of dark, dangerous and dirty places."
Dr Bomphrey is using his EPSRC-funded Fellowship to pursue this research. The fundamental aim of the work is to explore how natural selection has impacted on the design of insect wings and how these designs have been affected by the laws of aerodynamics and other physical constraints. "Evolution hasn't settled on a single type of insect wing design," says Dr Bomphrey. "We aim to understand how natural selection led to this situation. But we also want to explore how manmade vehicles could transcend the constraints imposed by nature."
18/09/2009: Secrets of long-haul insect flight revealed
Scientists studying the flexible wings of insects have discovered what makes them so efficient compared to the rigid wings of manmade aircraft. Researchers at Oxford University and the University of New South Wales first used high-speed video cameras to capture how locust wings change shape in flight, then computer modelling to reconstruct these shape changes in 3D, and finally ran simulations to discover how they deliver the sort of efficient flight that enables tiny locusts to make inter-continental flights.
A report of their research is published in this week’s Science.
‘Until very recently it wasn’t possible to accurately model the wings of insects in flight – partly because they flap so fast and partly because their shape is very complicated,’ said Dr Graham Taylor of Oxford University’s Department of Zoology, an author of the paper. ‘But with faster cameras and our advanced 3D simulation technology we can now see that the reason locust wings are so efficient is that the wing’s complex, changing shape ensures that the air always flows smoothly over its surface, never becoming detached as it would with a flat wing. We showed that by channelling the airflow in this way insect wings produce about 50 per cent more lift than similar rigid wings.’
‘We always thought that insects, with their complicated wing structures full of twists, curves and ridges, must know something about aerodynamics that engineers don’t, now we know what that is,’ said Professor Adrian Thomas of Oxford University’s Department of Zoology, senior author of the paper. ‘It really is throwing down the gauntlet to engineers looking to build micro air-vehicles – telling them that if they want these tiny flapping aircraft to be efficient as well as powerful flyers then they will need to have wings that change shape just like an insect’s.’
In their study the researchers used images from multiple high-speed digital video cameras to reconstruct how the 3D shape of a locust's wing [the Desert locust: Schistocerca gregaria] changes in flight. They then used their 3D models in a computer simulation to predict the aerodynamic flow generated by the flapping wings, and when the flow predicted by the model (Fig. A) was compared with experimental measurements from real insects it was an excellent match.
The team then re-ran their simulation with two simplified wing designs: In the first design they removed the wrinkles and curves but left the twist (Fig. B) , while in the second design they replaced the wings with rigid flat plates (Fig. C). The results were clear: the simplified models produced plenty of lift, but were much less efficient, requiring much more power for flight. The reason was that the complex deforming shape of the real wings allowed the air to flow smoothly over their surface, even as the wing was being flapped about. When the details of the real wings were lost, the flow separated away from the surface, resulting in high but costly lift. The consequences were dramatic: the fully deforming wings generated one and a half times the lift of the flat plate wings for the same amount of power.
Professor Adrian Thomas said: ‘The so-called `bumblebee paradox' - claiming that insects defy the laws of aerodynamics - is dead. Modern aerodynamics really can accurately model insect flight.’
For more information contact Professor Adrian Thomas on +44 (0)1865 271208 or email adrian.thomas@zoo.ox.ac.uk
Images and video of the locust flight modelling are available at: http://www.ox.ac.uk/media/news_releases_for_journalists/090915.html
Alternatively, contact the University of Oxford Press Office on +44 (0)1865 283877 or email press.office@admin.ox.ac.uk
Notes for editors
1. A report of the research, ‘Details of insect flight design and deformation enhance aerodynamic function and flight efficiency’, is published in Science, embargoed until 19:00 BST Thursday 17 September 2009.
2. The research was undertaken by Professor Adrian Thomas, Dr Graham Taylor, Dr Simon Walker and Dr Richard Bomphrey of the Oxford Animal Flight Group, part of the Department of Zoology at the University of Oxford, who are co-authors of the paper in Science, in collaboration with Dr John Young of the University of New South Wales.
3. This research was supported by EPSRC grant GR/S23049/01. The research leading to these results has also received funding from the European Research Council under the European Community’s Seventh Framework Programme (FP7/2007-2013)/ERC grant agreement no. 204513.
13/05/2009: Hoverflies' bendy wings raise a flap
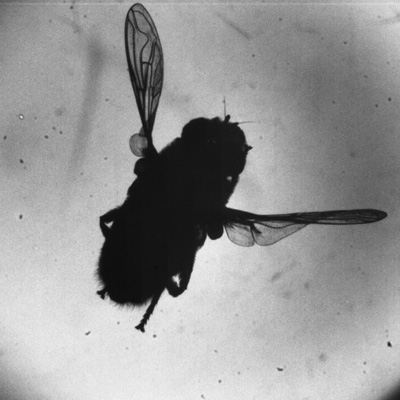
You would be justifiably alarmed to see an airliner’s wing twist through 45 degrees, but the flapping wings of a hoverfly deform like this 300 times every second. Add to this a large flap which flips up at right angles to the rest of the wing during manoeuvres, and you have what might be termed an “unconventional” configuration. With the aid of lasers and high-speed cameras filming at 4000 frames per second, Oxford scientists have begun to unravel the hoverfly’s secrets by reconstructing how the wings' three-dimensional shape changes through the stroke. Intriguingly, the hinged flap at the base of the wing seems to be intimately involved in the hoverfly's extraordinary manoeuvering performance. Aircraft designers are unlikely to stop using rigid wings anytime soon, but for small and highly manoeuvrable micro-air vehicles, bendy wings might just be the next big thing.
*For more information contact Dr Graham Taylor on +44 (0)1865
271219; or email graham.taylor@zoo.ox.ac.uk*
Notes for Editors:
- A report of the research, ‘Deformable wing kinematics in free-flying hoverflies’, is
published online in Journal of the Royal Society: Interface.
- The research was undertaken by Dr Simon Walker, Professor Adrian Thomas and Dr Graham Taylor of the Oxford Animal Flight Group, part of the
Department of Zoology at the University of Oxford.
- Dr Taylor is a Royal Society University Research Fellow and RCUK Academic Fellow. This research was supported by EPSRC grant GR/S23049/01. The research leading to these results has also received funding from the European Research Council under the European Community’s Seventh Framework Programme (FP7/2007-2013)/ERC grant agreement no. 204513.
03/05/2009: Bumblebee flight a triumph of power over finesse
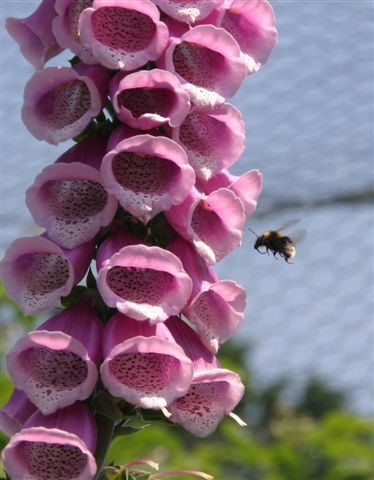
Brute force rather than aerodynamic efficiency is the key to bumblebee flight, Oxford University scientists have discovered.
In recent years scientists have modelled how insect wings interact with the air around them to generate lift by using computational models that are relatively simple, often simplifying the motion or shape of the wings. ‘We decided to go back to the insect itself and use smoke, a wind tunnel
and high-speed cameras to observe in detail how real bumblebee wings
work in free flight,’ said Dr Richard Bomphrey of the Department of
Zoology, co-author of a report of the research published in Experiments
in Fluids. ‘We found that bumblebee flight is surprisingly inefficient – aerodynamically-speaking it’s as if the insect is ‘split in half’ as
not only do its left and right wings flap independently but the airflow
around them never joins up to help it slip through the air more easily.’
Such an extreme aerodynamic separation between left and right sets the
bumblebee [/Bombus terrestris/] apart from most other flying animals. ‘Our observations show that, instead of the aerodynamic finesse found in
most other insects, bumblebees have a adopted a brute force approach
powered by a huge thorax and fuelled by energy-rich nectar,’ said Dr
Bomphrey. ‘This approach may be due to its particularly wide body shape,
or it could have evolved to make bumblebees more manoeuvrable in the air
at the cost of a less efficient flying style.’ Professor Adrian Thomas of Oxford’s Department of Zoology, co-author of
the report, said: ‘a bumblebee is a tanker-truck, its job is to
transport nectar and pollen back to the hive. Efficiency is unlikely to
be important for that way of life.’
Observing insects in free – as opposed to tethered – flight is a
considerable challenge. The Oxford team trained bumblebees to commute
from their hive to harvest pollen from cut flowers at one end of a wind
tunnel. They then used the wind tunnel to blow streams of smoke passed
the flying bees, to reveal vortices in the air, and recorded the results
with high-speed cameras taking up to 2000 images per second. From these
images the team were able to visualise the airflow over flapping
bumblebee wings.The old myth that ‘bumblebees shouldn’t be able to fly’ was based on
calculations using the aerodynamic theory of 1918-19, just 15 years
after the Wright brothers made the first powered flight. These early
theories suggested that bumblebee wings were too small to create
sufficient lift but since then scientists have made huge advances in
understanding aerodynamics and how different kinds of airflow can
generate lift.
click here for movie of bees in the laboratory (1.9MB .avi)
click here for bee movie (2.3MB .mov)
* For more information contact Professor Adrian Thomas on +44 (0)1865
271208; or email adrian.thomas@zoo.ox.ac.uk*
*Alternatively, contact the University of Oxford Press Office on +44
(0)1865 283877 or email press.office@admin.ox.ac.uk*
Notes for Editors:
- A report of the research, ‘Smoke visualization of free-flying bumblebees
indicates independent leading-edge vortices on each wing pair’, is
published online in Experiments in Fluids. Go to: http://www.springerlink.com/content/v66l43x615201104/fulltext.html
- The research was undertaken by Dr Richard Bomphrey, Dr Graham Taylor and
Professor Adrian Thomas of the Oxford Animal Flight Group, part of the
Department of Zoology at the University of Oxford who are co-authors of
the paper in Experiments in Fluids.
- RJB is a post doctoral research fellow at St. Anne's College, Oxford, and was supported for this work by the BBSRC.
01/06/2008: Team begins work on €2M ERC Grant
Dr Graham Taylor is among fewer than 300 recipients of the European Research Council's prestigious Starting Grants. The overall success rate was just 3% for this scheme, which drew applicants from across the EU and its associated states. Taylor's €1.95M grant lasts for 5 years and is intended to consolidate his research team. "This grant will enable my team to make significant new progress in understanding the flight control systems of animals" says Taylor. "Within a few years, we hope to be applying the insights we gain from Nature to Engineering , developing new biologically-inspired systems for aerospace applications and beyond".
Taylor's team, comprising three post-doctoral research assistants, one PhD student and a technician began work on the new project today. Their research will follow three tracks. One project will model the sensorimotor control systems of various insects by measuring the flight forces they generate while flying tethered in a virtual-reality flight simulator. A second project will analyse the effects of aeroelasticity on flight manoeuvres by measuring the detailed wing kinematics and deformations of the same insects flying freely in a virtual-reality arena. A third project will use onboard wireless video cameras and instrumentation to make measurements of the wing, tail and body kinematics of free-flying birds of prey. Using engineering tools to analyse these data will allow the team to test evolutionary hypotheses about how the physics and physiology of flight have been co-tuned, with applications to the design of control systems for autonomous vehicles. Among other things, the team hopes to explain how insects exploit rich sensory input to achieve their extraordinary stability and manoeuvrability, how birds and insects exploit wing deformation when aircraft designers shy away from it, and how birds succeed in maintaining yaw stability without the aid of a costly and inefficient vertical tailfin.
Notes for Editors:
- The European Research Council (ERC) is a newly formed agency of the European Commission.
- A total of 9167 applications were received for the first round of ERC Starting Grants at the close of call in April 2007. See ERC press release.
- Graham Taylor is a Royal Society University Research Fellow and RCUK Academic Fellow in the Department of Zoology, Oxford. He also holds the Peter Brunet Fellowship in Biological and Human Sciences at Jesus College, Oxford.
- The Department of Zoology was one of only a handful of academic institutions to receive more than one ERC Starting Grant this year: anotherof the Department's Royal Society University Research Fellows, Dr Angus Buckling, was awarded a grant of c. €1M for his work on the genetic and ecological bases of host-parsite specificity. See press release.
06/04/2006: An eagle's eye view of flight
Have you ever wondered what an eagle's eye view of the world would look like? Researchers at Oxford University's Department of Zoology have, and are using the latest in miniature spy cameras–carried by the bird–to find out. Drs Graham Taylor and Adrian Thomas came across the idea of mounting spy cameras onboard an eagle while acting as scientific consultants for the BBC's pioneering television series Animal Camera. They and their team are now using the same techniques to monitor scientifically how a trained eagle moves its wings, body and tail in free flight and to see where it looks as it does so <1.4MB video clip>. "Birds achieve remarkable manoeuvrability" explains Taylor. "By recording how the position and shape of the wings and tail vary in free flight while simultaneously monitoring the bird's head and eye movements, we can work out what inputs and outputs the eagle's control system receives and sends. By linking these to the actual motions of the bird, we can begin to unravel how its control system functions". "This is the first time anyone has got a handle on the three-dimensional shape of the wing in flight" adds Thomas. "Measuring wing shape on museum specimens is one thing, but measuring how wing shape changes in free flight is quite another when it comes to understanding the aerodynamics". To get their data, the researchers spent a cold week in Denmark this march working with three-times Women's World Champion paraglider pilot Louise Crandal and her eagle "Cossack" who is trained both to carry cameras and to follow Louise as she flies. This arrangement will ulitmately give the researchers some influence over what the eagle chooses to do in flight. So far, the researchers have been able to show that the eagle keeps its view of the world steady by fixing its gaze on a distant point and then flicking its head quickly in the direction it wishes to turn. As the body turns, it slowly catches up with the head, which remains steady until its next flick. Much the same technique is used by pirouetting ballet dancers, who avoid getting dizzy by fixing their gaze as they spin–just part of the answer to how Cossack is able to combine the power and grace of a dancer in turns that would dizzy the rest of us. But the researchers aren't ready to let on all they have found just yet, and with the next round of fieldwork planned for june, there are certain to be more new findings around the corner–watch this space!
Notes for Editors:
- Research presented on 03/04/06 at the Society for Experimental Biology Annual Main Meeting, Canterbury, UK.
- Graham Taylor is a Royal Society University Research Fellow at Jesus College, Oxford. Adrian Thomas is a University Lecturer at Lady Margaret Hall, Oxford. Both are members of the Department of Zoology.
- Research funded by the European Office of Aerospace Research and Development (EOARD).
- Still image Copyright of Louise Crandal, 2006. Video Copyright of Oxford University, 2006. High resolution versions of both can be supplied on request. Please contact Graham Taylor to discuss licensing.
- Louise Crandal is a freelance journalist.
16/10/2003: One rule fits all, U=3fA, from Bumblebee to Blue Whale
The stately sweep of a swan's wing contrasts dramatically with a finch's frantic flapping. Such seemingly effortless flight is one of the most characteristic features of the proverbial way of an eagle in the air – birdwatchers even use wingbeat frequency to tell one species from another. But why do small birds flap so much faster than large ones? Research published in this week's Nature by Drs Graham Taylor and Adrian Thomas of Oxford University, and Dr Robert Nudds of Leeds University, UK, may just have provided the answer. It turns out that there is a constant relationship between cruising speed and flapping frequency, not just in birds, but also across bats, fish, dolphins, and even insects. "Our wind tunnel experiments with insects and flapping models had led us to expect that there might be a rule that applies to flapping flight" explains Taylor, "but when we looked at all the available data, we were surprised to find that not only birds, but also bats, moths, locusts, and even dolphins and fish obeyed the same rule". The explanation, it seems, lies in the aerodynamic and hydrodynamic mechanisms that animals use to swim and fly. It's all a matter of timing and geometry. Flap too slowly and the wing will shed the swirling mass of air that generates the flight force before the end of the stroke, wasting valuable seconds of force production. Flap too fast and the wing will collide with the messy wake it leaves behind. Get the ratio right, and the wing will operate at a clean angle to the flow, trailing a neat, efficient wake that averages a jet to propel the animal forward. "Remarkably, the ratio of flapping speed to flight speed could vary more within a single bird forced to fly at a range of different speeds", says Taylor, "than across all 42 species of bird, bat and insect we looked at in cruising flight". The reason is simple: because flapping frequency varies so little, peak aerodynamic efficiency is only achieved over a narrow range of speeds, so animals sensibly tune their wingbeat frequency for peak efficiency when cruising - just like a well-designed car. The result is a stunningly simple rule of thumb: U =3 fA . Cruising speed ( U ) is roughly three times flapping frequency ( f ) times wingtip sweep amplitude ( A ). Extrapolating a little, this means that a blue whale sweeping its tail through a distance of 10m once every 5 seconds will cruise at 6m/s (20kmh) - too slow, sadly, to escape a whaler. At the opposite extreme, a bumblebee flapping its wings through 2cm, 140 times every second, should commute from its hive at 8.5m/s - remarkably fast for its size, but spot on what they actually achieve. 16th October 2003
Notes for Editors:
- Research published October 16th 2003 in the journal Nature (425, 707-711).
- Graham Taylor is a Weir Junior Research Fellow at University College, Oxford. Adrian Thomas is a University Lecturer at Lady Margaret Hall, Oxford. Robert Nudds is a post-doctoral researcher at Leeds University.
- Research funded by the Biotechnology and Biological Sciences Research Council and the Royal Commission for the Exhibition of 1851.