Exoplanet Atmospheres
Research Interests
My research is driven towards the discovery and characterization of potentially habitable worlds. We live in an exciting era which will see the construction of powerful instruments, such as the extremely large and giant segmented mirror telescopes, and will allow us for the first time in human history to directly answer the question: are we alone in the Universe? In the meantime, there is much to prepare, and my past and current research has focused on the discovery of exoplanets, the characterization of their atmospheres, and on precisely constraining models that describe the evolution of their host stars. Below you can read more about some of the projects I am currently involved with.
Exoplanet Atmospheres
Developing observational techniques that are capable of remotely and unambiguously identifying the constituents of an exoplanet atmosphere is key for hunting for signs of life in habitable worlds in the future. I have been working towards this by probing the atmospheres of hot Jupiters with ground-based techniques using high-resolution infrared spectroscopy, and I recently announced the detection of water in the atmosphere of the hot Jupiter HD 189733 b with this method. Although the detection of water is certainly not an indicator of habitability for this extremely hot planet, it is a promising step towards the robust detection of relatively complex molecules in the atmospheres of exoplanets using ground-based observations. The technique we used to find the water was first tested by the Leiden Exoplanet group in 2010, when Snellen et al used high-resolution spectroscopy to detect carbon monoxide high up in the atmosphere of the hot Jupiter HD 209458 b during one of its transits. The method works by individually resolving the forest of spectral lines in molecular bands in the planet’s spectrum. The planet is observed for about a tenth of its orbit during which the planet’s spectrum is significantly Doppler shifted (~km/s compared to the stellar lines ~m/s). This means the planet’s radial velocity curve can be separated from the static spectral features of the Earth atmosphere. By cross-correlating with a grid of model exoplanet atmospheres, we can identify specific molecules in the planet’s atmosphere and constrain how its temperature varies as a function of altitude i.e. if there are similar features to the Earth’s stratosphere for example.
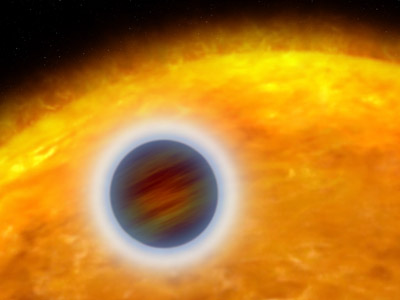
Low-Mass Stars
Stars with less than about half the mass and radius of the Sun, the so-called M-dwarfs, account for more than 70% of the stars in the Milky Way. Their small size and cool temperature make them the best targets for exoplanet surveys hunting for nearby habitable planets. This is because their favourable planet/star radius ratio results in deeper transit signals and larger radial velocity variations than their Solar-type brethren, and because their habitable zones are much closer in, leading to more transits per year. However, despite all this, M-dwarfs are severely lacking in stringent observational constraints, especially at young, pre-main sequence ages. Furthermore, there is a worrying and significant discrepancy between models of M-dwarf stellar evolution and directly observed measurements of their fundamental properties such as mass, radius, luminosity, composition (metallicity), and temperature, at all ages. This has important consequences for characterizing their planetary companions, where even small errors on the stellar host properties can significantly change the implied nature of its planetary companions and their potential for habitability.
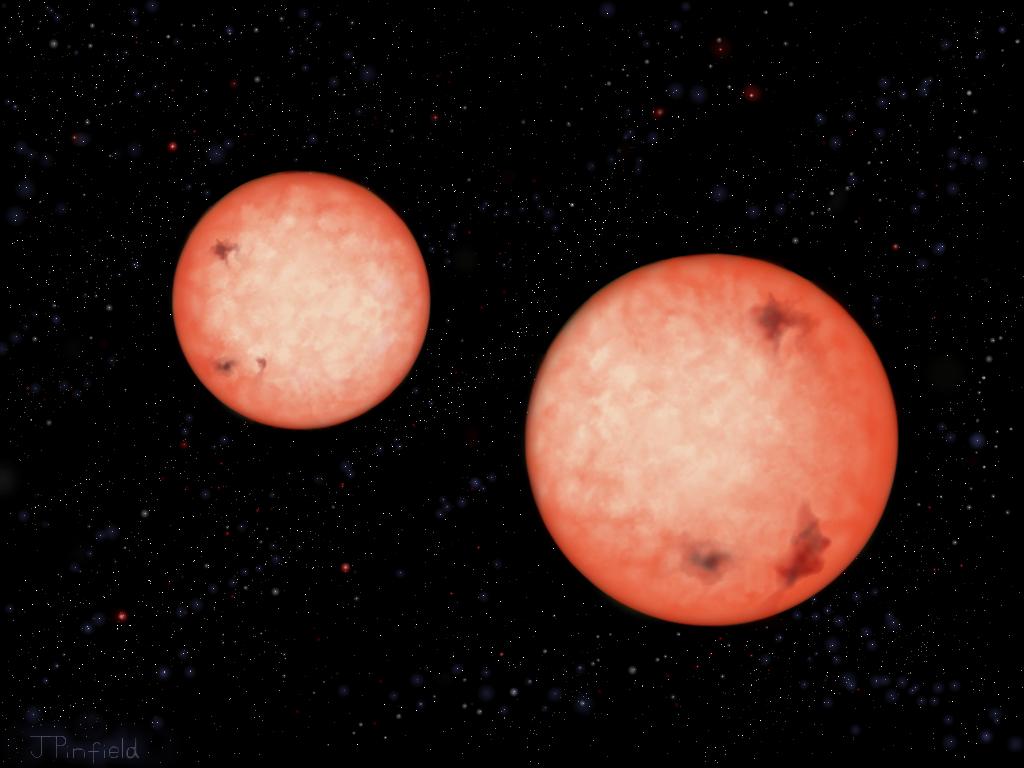
My research focuses on increasing the number of observational constraints on the fundamental properties of M-dwarfs. Using light curves and radial velocity curves of M-dwarf eclipsing binary stars (MEBs), I derive accurate and precise measurements (to the ~3% level) of the masses and radii of the component stars across a range of stellar ages (if you want to know the ins-and-outs of how this works, check out Chapter 2 of my thesis for a review). To first find these systems, I use two large-scale, ongoing, wide-field (near-)infrared photometric monitoring surveys: i) the WFCAM Transit Survey (WTS), and ii) the Monitor Project.
The WTS is a 200-night J-band survey of ~6,000 M-dwarfs in four 1.5 sq. deg regions of the sky. It acts as a poor weather back-up program for the Wide-Field CAMra (WFCAM) on the United Kingdom InfraRed Telescope (UKIRT) on top of Mauna Kea, Hawaii, and is designed such that one of its field is always visible for observing. The WTS has its first light on 5th August 2007 and is supported by the Marie Curie Initial Training Network RoPACS (Rocky Planets Around Cool Stars). My main findings from the WTS are i) the discovery and characterization of multiple MEBs, and a demonstration that magnetic fields cannot fully account for discrepancies between stellar evolution models and observations, and ii) the discovery, confirmation, and characterization of two new hot Jupiters, WTS-1 b and WTS-2 b.
The Monitor Project is a survey of nine young open star clusters. It aims to constrain low-mass stellar evolution at young ages by measuring the masses and radii of MEB cluster members. Given that most stars are expected to form in similar clusters, the Monitor Project also aims to assess the impact of their dynamical environments on planet formation. Check out Chapter 3 of my thesis to see my research on hunting for exoplanets and measuring MEBs in the ~100 Myr year old cluster M50 (NGC 2323).